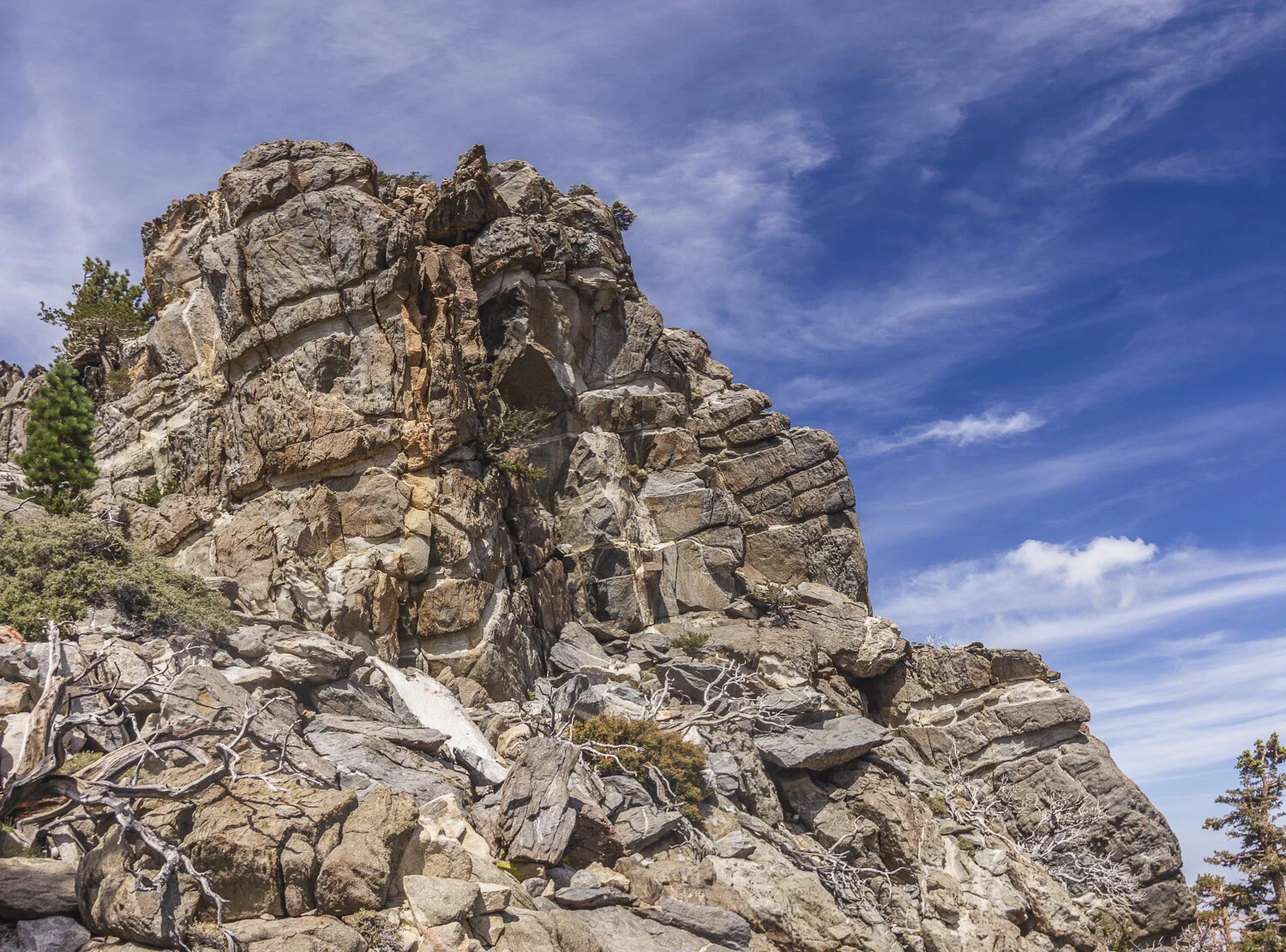
Geology of the San Jacinto Mountains
Cactus to Clouds: Mt San Jacinto and the Transformation Of The American West
10,834 feet above sea level: the majestic summit of Mt San Jacinto dominates the western horizon of the Coachella Valley, rising precipitously 2 miles above the desert sands of Palm Springs. Mt. San Jacinto is the highest peak at the very northern tip of the 900 miles long Peninsular Ranges reaching to the south end of Baja California.
For several hundred million years, mostly during the Paleozoic Era the western margin of North America was what geologist call a passive margin, simply defined as a continental edge where nothing much is happening tectonically, that is, no mountain building, subduction or volcanism. That’s like the east coast of North America today. Mostly all that is going on there, geologically, is weathering and erosion of existing uplands and deposition of their sediments in low lying areas and adjacent seas. Today, the evidence of those ancient sedimentary processes at the western passive margin are the stunningly dramatic landscapes of the Colorado Plateau. There, layer upon layer of sediment such as sandstone and limestone, thousands of feet thick, have been lifted from near sea level, where they were deposited over hundreds of millions of years, to many thousands of feet above sea level! As that was occurring, simultaneous weathering and erosion have created some of the most beautiful and awesome topography on the planet such as the Grand Canyon of The Colorado, Monument Valley, and Arches, Canyonlands, Capital Reef, Bryce Canyon and Zion National Parks and more!
What happened from those ancient times of passive deposition to create the landscapes of today, including Mt. San Jacinto of the Peninsular Range, the Sierra Nevada, the Basin and Range Province of myriad interior mountains and intervening desert basins, the Colorado Plateau and, indeed, the Rocky Mountains?
Everything began to change when the super continent of Pangea began to break up about 200 million years ago into separate and massive pieces of the earths crust called tectonic plates. One of those plates, we now know as the North American Plate, began rifting away from the Eurasian Plate, traveling westward. It was the birth of the Atlantic ocean. The western passive margin, as described above, began to overrun the eastern edge of the Farallon oceanic plate of the Pacific Ocean and geologic pandemonium broke loose. Volcanism, earthquakes and mountain building began along the western margin of North America. Over the following millennia the landscape was utterly transformed from the Rocky Mountains to the Pacific Ocean.
What follows is a necessarily brief history describing some of the major tectonic events that have created the modern landscapes of the American west with particular attention to the geomorphic provinces of southern California and the Coachella Valley. The processes continue to this day as evidenced by the ever present threat of catastrophic earthquakes and the great and still active volcanoes of the Cascade Ranges in the Northwest.
THINKING LIKE A GEOLOGIST
By Ted Konigsmark, GEOLOGIC TRIPS, Sierra Nevada
Most of us deal with common objects that we can see, measure, feel, and count. We think in terms of inches, feet and miles. We think in terms of seconds, minutes, hours, days, weeks, years, and centuries. We think in terms of ounces, pounds and tons, and in terms of hard and soft, hot and cold. However, to understand the geologic processes (that have formed the Sierra Nevada) you need to think like a geologist. This thinking is not difficult -just different. Here are some guidelines.
Geologic Time
A century may seem like a long time. However, geologists think in terms of millions and hundreds of millions of years. When you think in millions of years, enormous changes can take place even with very slow geologic processes. If you compare the 4.6-billion-year age of the earth to the age of a l00-year-old man, the oldest rocks in the Sierra Nevada were formed when he was 90 years old. The granite was intruded into the core of the mountains when he was 98 years old , and uplift of the Sierra block occurred about a month before his l00th birthday. A geologist must think in years the way an economist thinks in dollars—millions and billions.
Thinking Big
A mountain range such as the Sierra Nevada may seem large, and the rocks that make up the mountains may appear hard and brittle. Indeed, granite, limestone, and many of the other rocks of the Sierra are used as building stones. However, at a scale of miles rather than inches and feet, these seemingly strong and brittle rocks can easily bend and break. One must think big, and recognize that the rocks that make up the earth behave in a different manner when viewed on a large scale. Although upward and downward movements of the earth's surface of 30 or more miles may seem large, these movements are not large when compared to the size of the earth. If the earth were the size of a basketball, an uplift of 30,000 feet on the earth's surface would be equivalent to the thickness of a sheet of paper on the surface of the basketball, a slight imperfection on the basketball.
Living on a Dynamic Earth
Although the earth may seem like a large chunk of hard rock, it is quite different. The earth is dynamic.
The rocks that make up the earth are constantly moving. Parts of the earth's crust are being uplifted and other parts are subsiding. Some continents are being torn apart and others are colliding. The movement of the continents is caused by heat that is churning and moving the rocks deep within the earth. But why are these rocks so hot?
The only cool rocks on earth are those within a few miles of the surface. From the surface, the rocks get hotter with depth.
At a depth of about 60 miles, the rocks are so hot that they behave like warm plastic and flow at very slow rates. This heat is generated by decay of radioactive elements within the rocks.
Since rocks are not good conductors of heat, the heat builds up deep in the earth. The inner solid core and outer fluid core of the earth consist of iron and nickel and are extremely hot. The inner core and outer core are in motion, and rotate at a slightly different rate from the overlying mantle. The plastic-like rocks in the mantle are also in motion, and circulate in huge convection cells that reach from the core of the earth to the crust. These convection cells move the overlying brittle crust, tearing the crust apart over rising currents and carrying the crust downward into the mantle along descending currents. As the crust moves, new mountain ranges are formed in some places, and new ocean basins in other places. The geologist sees the oceans and mountains as temporary. Fortunately, these changes take place slowly. If you watched the surface of the earth in fast-forward on a VCR, you would need a seat belt, and you would be in for a wild and unexpected ride.
Erosion and Deposition
Most rocks are formed deep within the earth where the temperature and pressure is very high. When these rocks are uplifted and exposed at the earth’s surface, they are suddenly attacked by rivers, waves, plants, animals, ice, wind and rain. The rocks are also altered chemically. Some minerals combine with the oxygen and hydrogen at the surface to form new minerals. Other minerals simply do not like the low temperatures and pressures at the surface, and change into minerals that are stable at surface conditions.
Although humans like the surface of the earth, most rocks are uncomfortable at the surface and rapidly break down in this harsh environment.
When rocks break down by mechanical and chemical processes at the earth's surface, they form smaller clastic fragments - boulders, pebbles, sand, silt, and clay.
Rivers carry these sediments to low spots on the earth's surface and deposit the sediments in sedimentary basins. Over millions of years, entire mountain ranges can be worn down by weathering and erosion, until the mountain range is worn to a flat surface near sea level. The sediments that were eroded from the mountains accumulate in sedimentary basins. Sedimentary basins tend to subside as the sediments accumulate, and each new layer of sediment is piled on top of the previous layer of sediment. Over time, piles of sedimentary rocks can accumulate in these basins to thicknesses of tens of thousands of feet. The Great Valley is a large sedimentary basin that has accumulated thousands of feet of sediments that have been eroded from the Sierra Nevada.
Reconstructing the Past
When looking at the rocks exposed in a roadcut, on a mountainside, or along a river, try to erase, in your mind, the present landscape. When the rocks were formed, the landscape was completely different.
Look at the rocks and let them tell you what the landscape was like when the rocks were formed. If you are looking at limestone formed in some tropical sea, imagine yourself floating in the warm water of that ocean.
If there are several different types of rocks in an outcrop, each type of rock may tell a different story. The rocks changed as the landscape changed. To understand the geologic history of the area, the geologist must piece these stories together, like reading an old tattered book with many missing pages.
The Evolving Landscape
The landscape that we see today - the hills, peaks, lakes, sea cliffs, and valleys - are formed from rocks. These rocks are being attacked by various erosional and weathering processes. The shape of the landform depends on the type of rock that is exposed and on how the present weathering and erosion processes are working on the rock. When you look at a mountain, lake, or valley ask yourself several questions. What kind of rock is responsible for the feature? Are the rocks hard or soft? Are they fractured or massive? Are they being attacked by ice, rain, wind, or by chemical weathering? How is the landform changing? What will it look like in a short time - say a few thousand years? See, now you are thinking like a geologist.
Konigsmark, Ted, 2007, GEOLOGIC TRIPS, Sierra Nevada, GeoPress, Mendocino, CA, ISBN 0-9661316-5-7
First we need to appreciate the span of geologic time. The numbers on the right are in millions of years.
Plate Tectonics Summary
• In the early 1900s Alfred Wegener set forth his continental drift hypothesis. One of its major tenets was that a supercontinent called Pangaea began breaking apart into smaller continents about 200 million years ago. The smaller continental fragments then "drifted" to their present positions. To support the claim that the now-separate continents were once joined, Wegener and others used the fit of South America and Africa, the distribution of ancient climates, fossil evidence, and rock structures.
• One of the main objections to the continental drift hypothesis was the inability of its supporters to provide an acceptable mechanism for the movement of continents,
• The theory of plate tectonics, a far more encompassing theory than continental drift, holds that Earth's rigid outer shell, called the lithosphere, consists of seven large and numerous smaller segments called plates that are in motion relative to each other. Most of Earth's seismic activity, volcanism, and mountain building occur along the dynamic margins of these plates.
• A major departure of the plate tectonics theory from the continental drift hypothesis is that large plates contain both continental and ocean crust and the entire plate moves. By contrast, in continental drift, Wegener proposed that the sturdier continents "drifted" by breaking through the oceanic crust, much like ice breakers cut through ice.
• Divergent plate boundaries occur where plates move apart, resulting in upwelling of material from the mantle to create new seafloor. Most divergent boundaries occur along the axis of the oceanic ridge system and are associated with seafloor spreading, which occurs at rates between about 2 and 15 centimeters per year. New divergent boundaries may form within a continent (for example, the East African rift valleys), where they may fragment a landmass and develop a new ocean basin.
• Convergent plate boundaries occur where plates move together, resulting in the subduction of oceanic lithosphere into the mantle along a deep oceanic trench.
Convergence between an oceanic and continental block results in subduction of the oceanic slab and the formation of a continental volcanic arc such as the Andes of South America. Oceanic-oceanic convergence results in an arc-shaped chain of volcanic islands called a volcanic island arc.
When two plates carrying continental crust converge, both plates are too buoyant to be subducted. The result is a "collision" resulting in the formation of a mountain belt such as the Himalayas.
• Transform fault boundaries occur where plates grind past each other without the production or destruction of lithosphere. Most transform faults join two segments of an oceanic ridge.
Others connect spreading centers to subduction zones and thus facilitate the transport of oceanic crust created at a ridge crest to its site of destruction, at a deep-ocean trench. Still others, like the San Andreas Fault, cut through continental crust.
• The theory of plate tectonics is supported by (1) paleomagnetism, the direction and intensity of Earth's magnetism in the geologic past; (2) the global distribution of earthquakes and their close association with plate boundaries; (3) the ages of sediments from the floors of the deep-ocean basins; and (4) the existence of island groups that formed over hot spots and that provide a frame of reference for tracing the direction of plate motion.
• Three basic models for mantle convection are currently being evaluated. Mechanisms that contribute to this convective flow are slab-pull, ridge-push, and mantle plumes. Slab-pull occurs where cold, dense oceanic lithosphere is subducted and pulls the trailing lithosphere along. Ridge-push results when gravity sets the elevated slabs astride oceanic ridges in motion. Hot, buoyant mantle plumes are considered the upward flowing arms of mantle convection.
One model suggests that mantle convection occurs in two layers separated at a depth of 660 kilometers. Another model proposes whole-mantle convection that stirs the entire 2900-kilometer-thick rocky mantle. Yet another model suggests that the bottom third of the mantle gradually bulges upward in some areas and sinks in others without appreciable mixing.
Earth Science, 11th Edition, Edward J. Tarbuck and Frederick K. Lutgens, Pearson Prentice Hall
The creation of the Peninsular and Sierra Nevada Mountains, Illustrated
Early Jurassic time, Volcanic Ancestral Sierra and Peninsular Ranges
By Cretaceous Period Volcanism Ceases in This Area
During the the subduction of the Farallon Plate over tens of millions of years a vast accumulation of magma was intruded into the earth’s crust. When the volcanism moved to the east as the subducting Farallon plate flattened, it shut off the production of magma at the original location. Now, huge volumes of magma remaining in the earth’s crust for hundreds of miles of the volcanic arc began to cool and crystalize. Meanwhile miles of overburden of crustal rock and volcanic mountains were slowly being removed by weathering and erosion, eventually exposing the rock of the crystallized batholiths below to a new environment, the atmosphere, where weathering and erosion continue! Notably, exposure to the surface elements didn’t begin until a mere 2 or 3 million years ago! Thus, the topographic Peninsular and the Sierra Nevada ranges are very young. Geologically, the rock is much older… at least 70 to 80 million years older!
For more comprehensive geology of the region click on the button below
